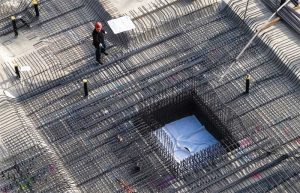
Reinforcing bar, commonly known as “rebar,” comes in the form of mesh, wires, or bars, and is designed to strengthen concrete under tension. Describing it in a more humanistic manner, one could consider rebar as a part of the muscular skeletal system of a building. Together, rebar and concrete work to resist tensile forces and increase the utility of a structure. Without rebar, people would not be able to build high into the sky or safely cross large expanses of water. However, the embodied carbon generated while creating and transporting traditional rebar is substantial. Luckily, new alternatives to traditional reinforcement are becoming more present in the market and the steel industry is making strides to build more sustainably.
Steel rebar
Historically, reinforcement has come in the form of steel. Common on all manner of job sites, the basic “black” bar is carbon steel rebar. It has high tensile strength, but corrodes easily, making it a poor choice in matrices with high likelihood of moisture exposure. ASTM A615M-09, Standard Specification for Deformed and Plain-Carbon Steel Bars for Concrete Reinforcement, covers parameters for lengths and coils of various reinforcement.
Epoxy-coated rebar is basically standard steel rebar with a thin coat of epoxy. It offers improved resistance to corrosion; however, the epoxy coating itself is delicate and chips easily during transport or installation. This can leave affected areas susceptible to corrosion. Guidelines for rebar with protective epoxy coatings are covered in ASTM A775M-19, Standard Specification for Epoxy-Coated Steel Reinforcing Bars.
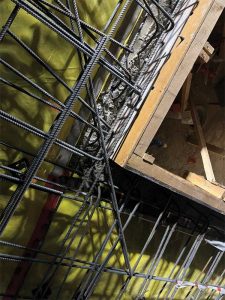
Galvanized rebar fills a similar role to epoxy-coated rebar, by way of the galvanizing process. Compared to epoxy-coated rebar, galvanized varieties are not as resistant to corrosion, but they are more difficult to chip and damage. The coating material for galvanized rebar is zinc, which is applied in various thicknesses through a process called “dipping” or “hot dipping.” Bolts, ties, dowel bars, and anchors can all be galvanized.
Stainless steel rebar has significant resistance to corrosion and does not require mitigating chipped areas like epoxy-coated rebar. This makes it an optimal choice for ease of installation and durability of the reinforced concrete. However, stainless steel reinforcement is traditionally very expensive and typically only found in projects which require long-term defense against corrosion, such as bridges or in geographies with higher seismic activity. It is also more embodied-carbon-intensive than standard rebar, by a margin of more than 10 percent. In North America, the main standard for stainless steel rebar is ASTM A955/A955M, Standard Specification for Deformed and Plain Stainless-Steel Bars for Concrete Reinforcement. It includes dimensional bar profiles and other information to assist project teams.
While steel provides excellent strength, its Achilles’ heel is its embodied carbon footprint. A ton of steel is worth about a ton of greenhouse gas (GHG) emissions, approximately equivalent to the amount of carbon dioxide (CO2) burned in 40 home barbecue propane tanks. Steel also has a higher embodied carbon footprint (by weight) compared to concrete. According to information from the World Steel Association, steel production equates to six to seven percent of global GHG emissions.
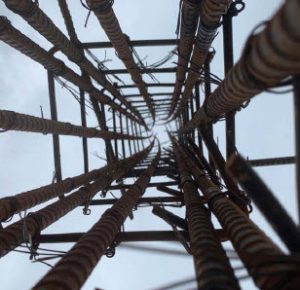
The main driver of steel emissions is the production process. There are two primary types of factories: the basic oxygen furnace (BOF) and the electric arc furnace (EAF). Steel is an alloy of iron and carbon. Legacy BOF furnaces burn fossil fuels to heat iron ore, coke, and limestone to temperatures exceeding 2000 C (3632 F). This is then mixed with 25 to 40 percent iron and steel scraps to make new steel. Modern EAF furnaces melt iron and steel scraps to create new steel, achieving much higher recycled content rates of 90 to 100 percent. While EAFs have become the predominant form of steel production in the U.S., BOFs are still the most common furnaces used around the world.
Given the high recycled content, steel from EAF production types tends to be about half as carbon intensive as BOF steel. However, reducing steel’s carbon footprint is not always as easy as specifying steel from an EAF factory, as production type is tied to steel shape. Hot rolled steel shapes, for example, are typically produced in EAF factories in the U.S. However, hollow structural section (HSS) shapes are more likely to come from BOF factories. Rebar can be produced using either BOF or EAF processes, thus sourcing rebar from EAF furnaces is preferred.
The efficacy of specifying higher recycled content as a method to reduce the embodied carbon footprint of steel has also recently been challenged. Since the amount of scrap steel available is limited, some experts suggest specifying a higher recycled content in metal products only arbitrarily changes the manufacturer’s allocation of accounting for scrap—shifting this benefit from one buyer to another, rather than creating any substantive change in supply chain practices. Until specifying higher recycled content starts to make steel deconstruction and recovery practices more desirable, and effectively adds more scrap to the system, it is challenging to create change through specifying higher recycled content.
Your article on rethinking rebar and green reinforcement methods is enlightening and timely. It offers valuable insights into sustainable construction practices, paving the way for a greener future in the industry.