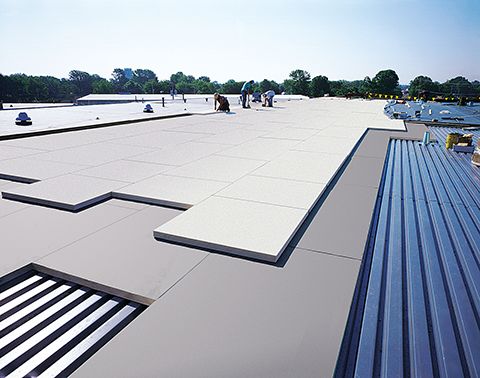
by James L. Hoff, DBA
With the release of the latest version of the Leadership in Energy and Environmental Design (LEED) rating program, the concept of ‘disclosure’ has taken center stage as the next big topic in the ongoing discussion of how sustainable buildings are defined and evaluated. Sharing the stage are new tools that have emerged to help measure the ‘greenness’ of building products.
Many different stakeholders within the building community have been active in the promotion of disclosure, but they all tend to share the same questions. A particular building material may help save operating energy, but how does it impact other equally important environmental concerns? A product may have a high recycled content, but after the effort required for salvage, transportation, and conversion is there still a tangible net environmental benefit? Beyond specific environmental concerns, how does the product affect the safety, health, and well-being of building occupants? Unfortunately, many of these questions cannot be answered effectively using current tools such as energy calculators and one-dimensional green product certifications.
Each of these questions is related to common concerns held not only by green building advocates but also the entire building design community: Do we have the kind of information about building products to make informed decisions? Do simplistic claims or categories of greenness help or hinder our analysis? Do current manufacturer data sheets and reports provide adequate information? Finally, do we understand exactly what goes into the building materials we use and how these ingredients may affect the well-being of building occupants?
EPDs and standardized processes
One of the newest tools to be integrated into the selection of sustainable building materials is the environmental product declaration (EPD). While almost everyone associated with the building envelope industry has become aware of this new tool, few industry stakeholders have had the opportunity to learn about the specifics of how they are developed and how they may be used.
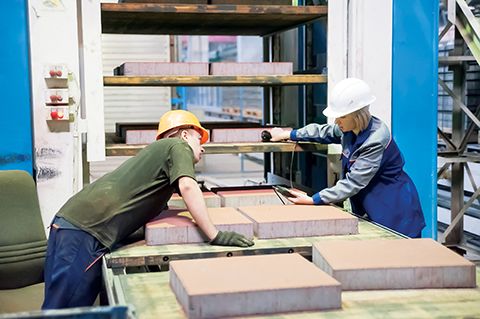
EPDs have been around the longest of all the new product disclosure tools, and the current procedures to develop EPDs have been in place for over a decade. However, the fact even the best-established disclosure tool is still relatively new attests to how quickly the concept of disclosure has entered the construction market. At the same time, the current EPD process has been built on a solid, science-based approach that examines total environmental impact over the entire life cycle of a product. There are many excellent definitions of EPDs, but for this article’s purposes, the EPD may best be described as a standardized process to disclose environmental impacts and other factors over the lifecycle of a product using quantifiable measures as applied to a common functional unit for similar products. This definition should be especially useful when broken down into its key elements.
As the environmental assessment of products has evolved, international standards have been developed to help ensure these practices are conducted in a consistent and reliable manner worldwide. In almost all cases, these procedures are based on standards adopted and maintained by the International Standards Organization (ISO). Standards related to the underlying practice of lifecycle assessment (LCA) are contained in ISO 14040, Environmental Management−Lifecycle Assessment: Principles and Framework, and ISO 14045, Environmental Management−Eco-efficiency Assessment of Product Systems: Principles, Requirements and Guidelines, which cover the key principles and framework as well as more detailed requirements and guidelines for completing the LCA process.
ISO standards related to the specific structure of EPDs are in ISO 14025, which covers the basic principles for applying EPDs to all kinds of products. Additionally, ISO 21930, Sustainability in Building Construction−Environmental Declaration of Building Products, contains additional principles for applying EPDs to building products.
The use of well-established procedures helps ensure the information disclosed in an EPD has been developed in an objective and scientific manner. In fact, the type of EPD required by LEED and other green building guidelines must be based on ISO standards and requires a final third-party review to validate the disclosure for accuracy and reliability.