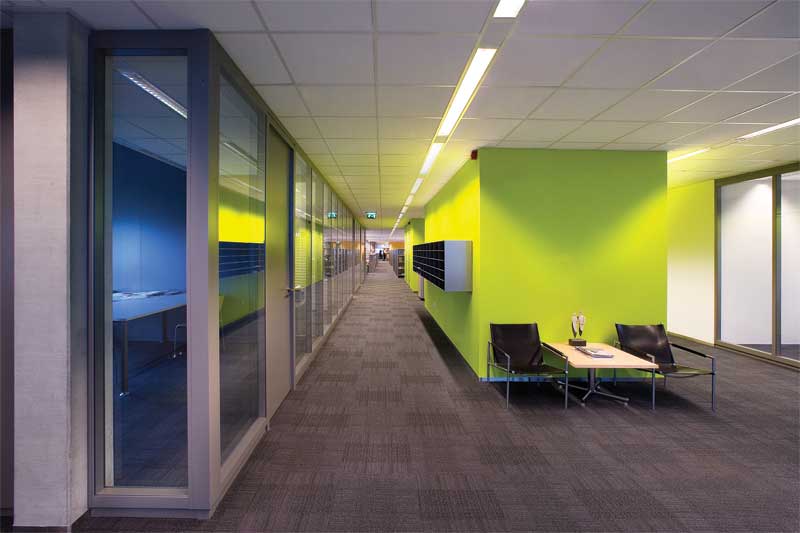
by Gary Madaras, PhD, Assoc. AIA
Sound isolation between rooms can be important for speech privacy. For example, in patient exam rooms in a medical building, conversations between patients and their doctors are meant to be confidential; similarly, discussions in an enclosed office between a human resources (HR) director and an employee are meant for no one else. Even when speech privacy is not a concern, sound transmitting from one room into another can be annoying or distracting and can inhibit productivity, concentration, and relaxation.
Achieving sound isolation between rooms relates to the overall construction of those spaces’ envelopes, including the walls, floors, windows, doors, and sometimes ceilings. The overall level of sound isolation often depends on the weakest link in the construction.
Acoustics requirements in building standards, guidelines, and rating systems list sound transmission class (STC) most frequently as the sound-isolation metric. STC requirements generally range from 40 to 50, with STC 45 being the most commonly occurring requirement for interior construction when sound isolation is needed. (For more, see this author’s “A Guide on the Four Categories for Acoustics Criteria in Building Standards and Guidelines,” which was published in the July−September 2016 edition of Acoustical Interior Construction.) Demising walls are required to be full-height—from structural floor slab to structural floor slab or roof, with any penetrations sealed airtight.
As a cost-saving measure in some buildings, interior walls are instead stopped at the height of a suspended, modular acoustic ceiling. They do not extend full-height up to the structural floor slab or roof. This practice not only saves on the construction cost of the walls, but also creates a continuous open space above the suspended ceiling that can be used as a return-air plenum.
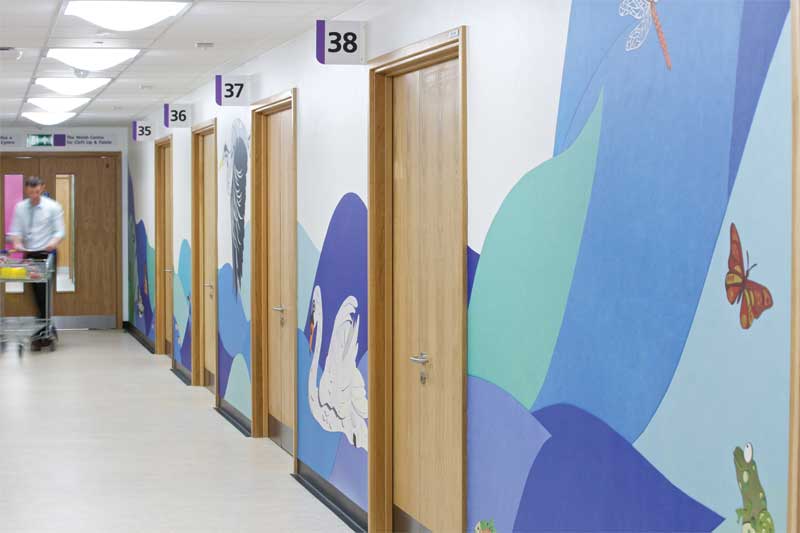
Specifying a plenum to return air can save money, compared with the cost of adding rigid metal return-air ductwork above the ceiling. This design approach typically does not comply with minimum sound-isolation performance requirements in standards, guidelines, and rating systems because sound can transmit at higher levels through the lightweight acoustic ceiling and over the partial-height wall. Some designers and building owners still elect to use this approach for the cost savings.
Effects of noise flanking paths
Eliminating the need to choose between a design approach that works acoustically and one that
works financially was one of the motivations for the Optimized Acoustics Research Program, an ongoing, multi-year, multi-organization investigation into cost-effective means of designing and constructing interior architecture that complies with the acoustic requirements in industry standards, guidelines, and building rating systems. (For more, visit www.optimizedacoustics.com. The current program includes manufacturers and a material testing laboratory; membership will vary in the future according to the topics being investigated. The initiative began in 2014, and progress updates of the findings, such as this article, have been presented at and published in the proceedings of InterNoise, NoiseCon, and Acoustics Week Canada, as well as published in Sound & Vibration and Canadian Acoustics.) An early phase of this initiative investigated the effects of noise flanking paths, or ‘leaks,’ through penetrations in the ceiling system when the demising walls stop at the height of the ceiling instead of extending full-height.
Suspended, modular ceiling systems typically have recessed light fixtures, open return-air grilles, supply air diffusers, and other miscellaneous penetrations for sprinkler heads, loudspeakers, security/surveillance devices, and Wi-Fi devices. These openings and penetrations in the ceiling system create noise flanking paths, through which noise transmits more easily between rooms.
what about security of the adjacent room in case the plenum barrier is used.