by Steven D. Marino
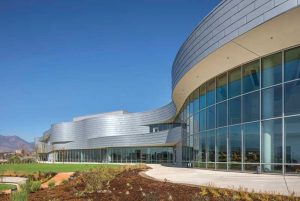
Its beauty can turn heads. From the functional to the fantastic, glass delivers on numerous levels in contemporary building designs—aesthetics, performance, quality, and energy efficiency, to name a few. It is no wonder architects make it the centerpiece of building designs. As the focal point of high-visibility structures, expansive glazing areas and large insulating glass units (IGUs) take more space on curtain walls, storefronts, and interior building components than ever before. Despite the numerous appearance and performance advantages, glass has a breaking point.
Primary causes of glass breakage
Although a relatively rare occurrence, the breakage of glass post-installation in high-rise buildings raises several questions beyond just the immediate need to replace the unit(s). Was it a one-time event, or part of a pattern of similar breaks in a particular glass product? Did the glass shatter into hundreds of pieces or simply crack? Was the glass properly installed? What role did weather play?
Although these questions are typically part of a more in-depth investigation, any incident always demands an answer to this fundamental question: Why did the glass break?
Like many brittle materials, glass, whose compressive strength is higher than its tensile strength, breaks or fails when stresses approach or exceed the material’s limits. Simply put, when an external force applied to a glass exceeds its maximum strength, the product fails.
A prevalent contributing factor of breakage is edge damage. Even minor defects on the edge of a glass panel can reduce its strength by more than 50 percent, compromising its ability to resist both thermal and mechanical loads.
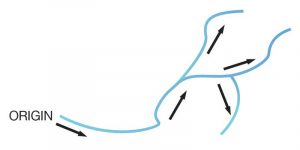
Images courtesy Vitro Architectural Glass
Although edge damage in and of itself does not break the glass—after all, the material may remain functional throughout its service life—it creates a weak point that may make the glass susceptible to conditions increasing the likelihood
of breakage.
In some instances, the cause of breakage can be traced to inclusions, which can be non-homogenized, or not fully melted, batch materials or contaminates such as nickel and aluminum. Two types of inclusions are nickel sulfide (NiS) and aluminum oxide (Al2O3) ‘stones.’
Many inclusions ‘behave’ the same way glass does—they expand in heat and contract in cold. Although most inclusions do not directly cause breakages, their size, composition, and location are the primary factors in the small percentage of inclusion-related incidents that actually occur.
Identifying the break origin
When there is a pattern of repeated glass breaks, it is critical to explore the reasons for such incidents. Typically initiated by the building owner or glazing contractor, this investigation should begin with identifying the origin of the break. This is possible only if pieces of the broken glass can be retained or re-assembled into the original orientation.
Identifying the break origin can provide hints about the following:
- mode of glass failure—is it mechanical or thermally induced stress;
- the stress or tension level at which the breakage occurred; and
- other contributing factors—were there digs (deep, short scratches) resulting from glass-to-glass or glass-to-metal contact, did a projectile hit the glass, or is there edge or surface damage?
To find the origin of a break, the first step is to assess its direction by inspecting the fracture lines, or ‘Wallner lines,’ in the glass. These rib-shaped marks, distinguished by a wave-like pattern through the thickness of the glass, begin at the break origin and radiate along break branches. The marks (or wave-like pattern) almost always concave in the direction from which the crack was propagating. The concave side of the wave is the origin and the convex section is the direction of propagation of the fracture.