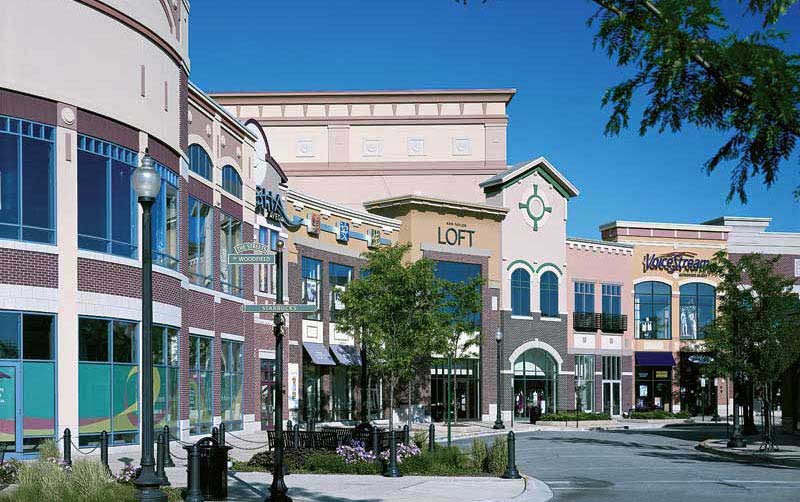
by J.W. Mollohan, CCPR, CEP, CSI
In light of the federal government’s goal of improving the energy efficiency of new and existing buildings, model energy codes are becoming more stringent. The effort to increase energy efficiency has been further emphasized by initiatives set forth by the 2009 American Recovery and Reinvestment Act’s State Energy Program, and a congressional mandate that all states, must comply, at a minimum, with the American Society of Heating, Refrigerating, and Air-conditioning Engineers (ASHRAE) 90.1, Energy Standard for Buildings Except Low-Rise Residential Buildings, no later than October 18, 2013. The most recent update to ASHRAE 90.1 is the standard on which the 2012 version of the International Energy Conservation Code (IECC) is based. The International Energy Conservation Code (IECC) establishes the requirements for building design, materials, and performance related to energy efficiency. Several successive iterations of IECC (2003, 2006, 2009, and 2012) are currently available for adoption by states and local jurisdictions. Currently, the most widely adopted is the IECC 2009 version. As IECC has become more stringent with each new release, it is crucial to be aware of the version in use where a project is to be located. To help determine this, the International Code Council (ICC) and the Department of Energy (DOE) maintain websites with the most recent adoptions.
Building enclosures and IECC
As might be expected, IECC gives considerable attention to the building enclosure’s insulation levels. The code divides the United States into eight Climate Zones and establishes specific requirements for the type, amount, and placement of insulating materials—cavity and continuous—in the exterior enclosure.
For exterior walls, the 2006 IECC requires both insulation in the stud cavity and continuous insulation (ci)—usually either board stock or sprayed polyurethane foam (SPF) outboard of the exterior sheathing for commercial steel-framed wall assemblies in Climate Zones 5 to 8. In 2009, IECC expanded the requirement for ci to include Climate Zones 3 and 4 and increased the required amount of ci in Zones 5 and 6. It now prescribes the use of exterior ci in nearly 90 percent of the country.
New design standards are also emerging, such as ASHRAE 189.1, Standard for the Design of High-performance Green Buildings. This standard includes prescriptive R-value cavity and ci requirements for commercial buildings in all eight Climate Zones. ASHRAE 189.1 has already been adopted by the U.S. Army Corps of Engineers (USACE) for federal and military construction.
The IECC gives design professionals multiple options to satisfy code requirements. Whole building modeling allows the user to examine potential solutions. The design professional may also either elect a prescriptive or performance path for determining the necessary amount of insulation in exterior wall assemblies.
The prescriptive method relies on the R-value of only the insulation materials and matches those values to predetermined insulation requirements for various wall types and Climate Zones. For example, the IECC establishes minimum prescriptive R-value for both cavity and exterior ci for steel and wood-framed wall construction based on each Climate Zone. If these values are met, no further analysis or calculations are required.
The performance method is slightly more complicated, but allows the design professional the flexibility to use knowledge and experience in creating the most appropriate solution to the clients’ needs, while still meeting code requirements. This method’s calculations are based on the wall’s U-factor (the fractional inverse of R-value [i.e. an R-value of 13 equates to a U-factor of 1/13, expressed by the decimal 0.0769]) and takes into account the thermal resistance rating of not just the insulation materials, but also all the wall assembly components, analyzed together to meet the Climate Zone’s thermal performance requirement.
The prescriptive and performance methods, though taking different approaches, generally produce similar results in the amount of both cavity and continuous insulation required for a particular Climate Zone and wall type. Perhaps a little insight into some of the problems the modern energy codes are attempting to solve may help when deciding which path best serves a project’s needs.
Making walls more efficient
The updates to the building energy codes are intended to address several critical issues with traditional building methods, specifically thermal bridging and the relative ineffectiveness of cavity insulation.
A typical framed wall assembly used wood or steel studs with some form of cavity insulation—usually fiberglass batts. Although economical, this assembly is thermally inefficient because only the cavities are insulated. The framing separates the insulation into non-continuous pockets and allows energy in the form of heat to conduct through the studs, bypassing the insulation and creating a condition known as ‘thermal bridging.’ Thermal bridges, along with convective losses, can reduce the effective performance of cavity insulation in steel-framed wall assemblies by 50 percent or more.
Among the independent resources reinforcing the reality of energy loss and reduced effective cavity insulation values due to thermal bridging, is a study conducted at the Department of Energy’s (DOE’s) Oak Ridge National Laboratory (ORNL). Performed to investigate the difference between the rated R-values of common wall designs and their tested effective value, the study evaluated “whole wall” construction, including materials and framing discontinuities such as transition details at windows, doors, roofs, floor lines, and typical wall-accessory penetrations. Though the claddings tested varied, all were mounted to the constant of a nominal 89-mm (3 1⁄2-in.) steel-framed wall assembly with 12.7-mm (1⁄2-in.) gypsum interior wallboard, R-11 fiberglass batts, and 12.7-mm glass-mat faced gypsum exterior sheathing. The claddings compared included:
- exterior insulation and finish system (EIFS);
- brick veneer;
- glass;
- stone and cast-stone masonry;
- stucco; and
- wood siding.
I think it’s good that there is more of an effort made to be more energy efficient. I’ve been in places where having good insulation isn’t possible, so it’s great that we have that luxury. Conserving energy is always a plus, because it’s more comfortable, and it’s more money efficient!