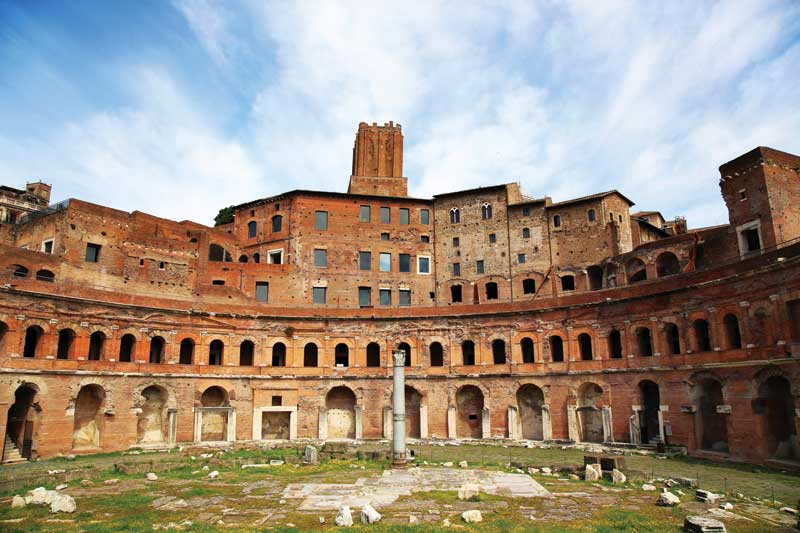
by Christopher Bennett, CSI, Assoc. AIA, and Rae Taylor, PhD
Carbon dioxide (CO2) is a naturally occurring gas byproduct of every organism that breathes oxygen; it is found deep in the oceans and high in the atmosphere. It is also vitally important—without CO2, photosynthesis would not be possible, and life would not exist. However, having too much of this greenhouse gas (GHG) can also bring harmful consequences directly affecting climate and humankind. Concrete, unfortunately, plays a role in this.
Carbon dioxide is created in various ways, from the decomposition of organic material to the natural rhythms of the oceans to seismic activity. Human-centered sources of CO2 include deforestation, the burning of fossil fuels, and—to a significant extent—the production of concrete. This may be unsurprising given the material’s scope—concrete is everywhere. As suggested by Bill Dubois in his October 2016 article for The Construction Specifier, “Healthy Concrete Systems,” the building product could be considered part of the muscular skeletal structure supporting the entire built environment. (See The Construction Specifier article by Bill DuBois and Christopher Bennett, “Healthy Concrete Systems: Defending Design Intent,” which appeared in the October 2016 issue. Visit www.constructionspecifier.com/healthy-concrete-systems-defending-design-intent.) Concrete is in our homes and in our schools; it spans waterways in the forms of bridges and connects destinations with endless miles of road.
Concrete is the most widely used manmade building material in the world. It is relatively inexpensive to manufacture, and basic concrete construction is easy to teach. The material’s convenience propelled it to its top position as a building product, while its strength and versatility has kept it there despite obvious contributions to total CO2 emissions. There is no likely contender for a substitute to concrete on the horizon—replacing concrete as a building technology simply is not an option. That said, there are ways to reduce its environmental impacts.
Concrete manufacturing
The manufacturing process of concrete, with emphasis on cement, has been under pressure to be greener for decades. Currently, fuel consumption in kiln-fired production of Portland cement, along with CO2 emission during calcination of carbonaceous rock and use of cement, uses a significant amount of energy and produces large amounts of GHG emissions. In 2010, 3.2 billion tons of CO2 were emitted to the atmosphere from the production of 3.9 billion tons of cement. Going forward, cement production is expected to grow each year by about 2.5 percent, potentially reaching 4.4 billion tons by 2050. Without any significant changes to how the global construction industry approaches concrete, the planet will continue to be burdened with additional CO2.
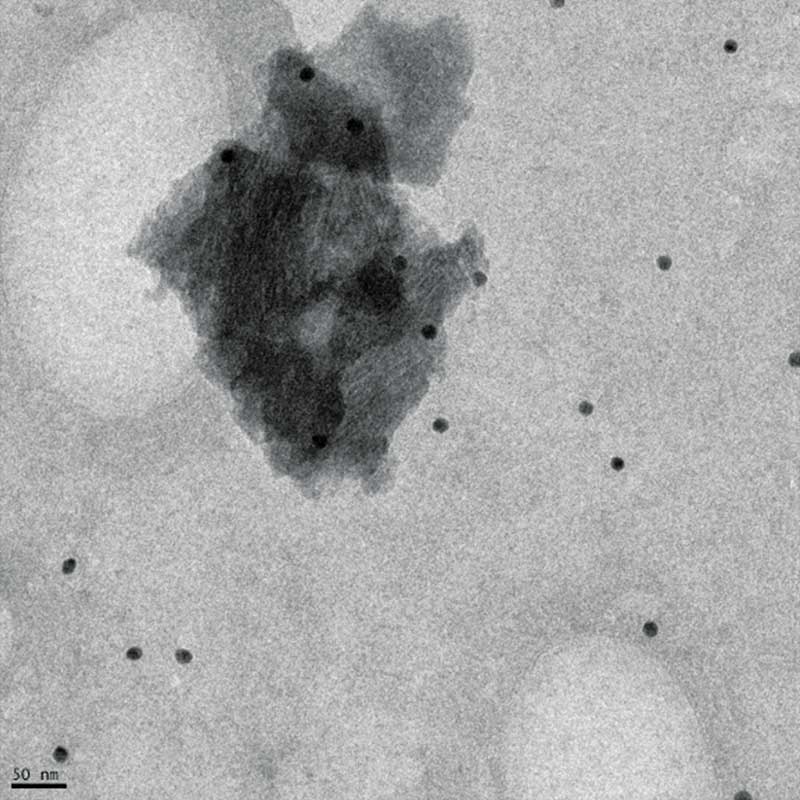
Photo courtesy Rae Taylor
Many countermeasures, such as improving the thermal efficiency of kilns and cooler systems, or using alternative fuels rather than carbon-based ones, have already been put in place, but with limited effect. While it is possible to make further advancements, the industry seems reluctant for numerous reasons, including:
- fluctuating fuel availability;
- increased atmospheric pollutants (See the Pembina Institute and Environmental Defence’s 2014 article, “Alternative Fuel Use in Cement Manufacturing.” It can be downloaded from www.pembina.org/pub/alternative-fuel-use-in-cement-manufacturing); and
- need for additional financial incentives.
The solution seeming to have the most direct promise of a positive effect would be replacing ordinary Portland cement (i.e. the main culprit of CO2 release) with another material still providing strength. This suggestion is not a new one—as early as the 1960s, the potential of ground granulated blast furnace slag (GGBS) as a cement replacement material was discovered. (See H.F.W. Taylor’s Cement Chemistry [2nd edition, vol. 2], published in 1997 by Thomas Telford, for more on Locher’s 1966 work.) Earlier than this, fly ash was known to have similar properties (“Properties of cements and concretes containing fly ash,” by R.E. Davis et al, was published by the American Concrete Institute [ACI] in 1937.), even though the reason was not fully understood until more recently. (See R. Taylor et al’s “Composition and Microstructure of 20-year-old Ordinary Portland Cement-ground Granulated Blast-furnace Slag Blends Containing 0 to 100% Slag,” published in Cement and Concrete Research [40(7)] in 2010.)
Before exploring alternatives to Portland cement, it is important to understand this ingredient’s role in concrete. When mixed with water, cement undergoes an incredibly complex reaction; it produces a variety of compounds depending on its original composition. Of these, the most important is calcium silicate hydrate (C-S-H)—the ‘glue’ holding the concrete together. Without C-S-H, one would simply have a loose collection of sand and stone—certainly nothing resembling the Three Gorges Dam. Construction workers used some 16 million m3 (21 million cy) of concrete in this structure.