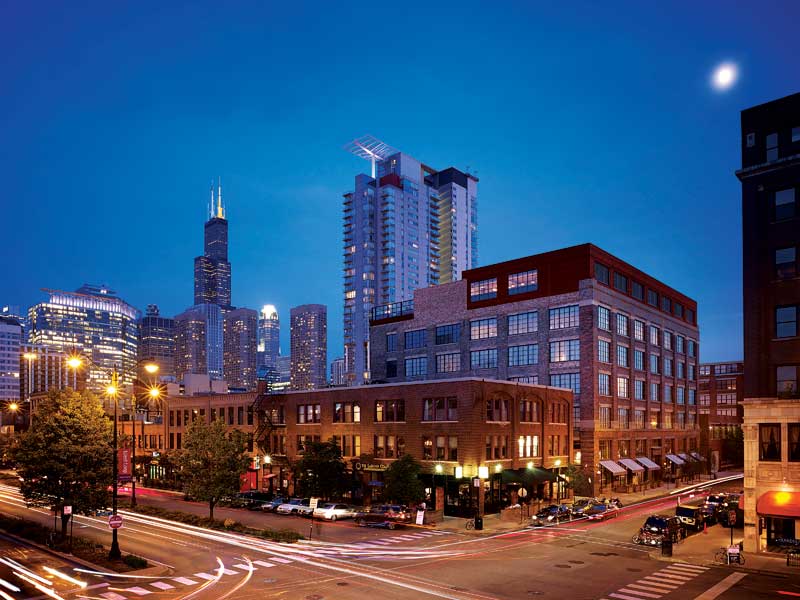
by Steve Fronek, PE
By comparing exterior noise levels with interior requirements, and considering background noise levels, a project’s required level of window or curtain wall acoustic performance can be inferred either in specific frequency bands or by using one of the single-number rating systems described in this article.
Sound pressure level and frequency
Proper acoustic design begins with an understanding of the fundamentals of sound.
Sound pressure level
Sound pressure level (SPL) is a common sound measurement typically expressed in decibels (dB). A decibel is a logarithmic ratio of the pressure of the measured sound to a reference pressure. Normal conversational speech is approximately 60 dB.
The threshold of human hearing, or the smallest audible pressure level, is usually assumed to be
20 µPa (20 x 10-6Pa). This is the reference pressure for calculating SPL, corresponding to an SPL of 0 dB.
The equation is:
SPL (dB) = 10 log10(p2/p2ref) = 20 log10(p/pref)
Where:
p = SPL in Pa (psi)
pref = reference SPL in Pa (psi) = 20 μPa (2.9 x 10−10 psi)
Sound is also characterized by frequency, which is measured in cycles per second or Hertz (Hz). Normal human hearing can sense frequencies from about 20 Hz to 20,000 Hz. This audible frequency range is often further divided into smaller octave bands (OBs) or third OBs, which are commonly denoted by their ‘center frequency,’ so the third OB centered at 31.5 Hz would correspond to a frequency range of 28.2 to 35.5 Hz. A full OB also is denoted by its center frequency. Therefore, the 31.5 Hz full octave corresponds to a range of 22.4 to 44.7 Hz.
Each full OB comprises a low-, middle-, and high-frequency third OB. The lower third OB of the 1000 Hz full OB is centered at 800 Hz, and includes the frequencies from about 708 to 891 Hz. Similarly, the middle third OB of the 1000 Hz full OB also is centered at 1000 Hz and includes the frequencies from about 891 to 1122 Hz. Finally, the higher third OB of the 1000 Hz full OB is centered at 1250 Hz and includes the frequencies from about 1122 to 1413 Hz.
Sounds in the range of 20 to 500 Hz tend to be categorized as low-frequency sounds. Sounds like rumbles and roars carry much more sound energy than high frequency squeaks and buzzes, and therefore, are more difficult to absorb. Similarly, low-frequency sounds are also heard more easily by typical human hearing than high frequency ones at the same distance from a given sound source.
Since the human ear is the most sensitive to midrange frequencies of 500 to 8000 Hz, sound-level meters usually include a filter to weight frequencies in a way that approximates human perception of loudness. This is called A-weighting; the A-weighted logarithmic sum of all such weighted frequencies is designated as dBA.
Generally, glass decreases sound transmittance at higher frequencies better than it does at lower frequencies. One should be especially cautious if acoustic expectations for windows or curtain walls dictate a certain performance level for any frequencies below 1000 Hz.