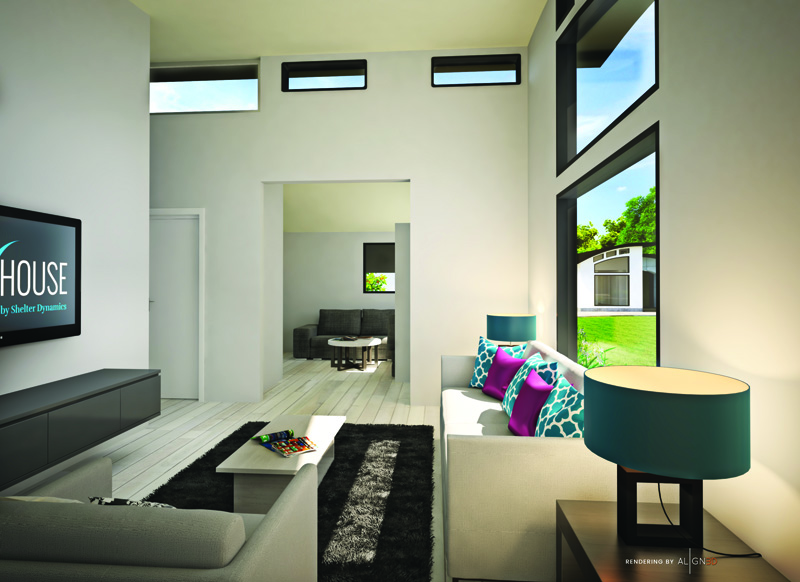
by Todd Sims
This year, ‘resiliency’ emerged in the building landscape as more than a buzzword. Many regions around the world are increasingly subject to the rigors of various impacts, including extreme weather, population shifts, disease, power or communication disruptions, and financial shocks. Urban and rural spaces alike require structures able to withstand volatile stresses while reducing the additional resources, time, and labor needed to rebuild and relocate.
Resilient structures necessitate innovative materials that can not only endure stress, but also return to a functioning, usable state. For example, a bridge is resilient if the materials used can expand and contract with cold weather, high winds, and changes in traffic patterns. Specific insulation materials can add strength to walls; they help eliminate the movement of air and moisture while increasing a wall’s resiliency.
Last year, the Resilience Building Coalition, which includes the National Institute of Building Sciences (NIBS), American Institute of Architects (AIA), CSI, and 37 other leaders of America’s design and construction industry, released its first progress report, introducing a set of principles to keep the conversations around resiliency going. (CSI joined the founding signatories as an ‘amplifier,’ committing to the advancement of the group’s goals. To read the statement, visit aiad8.prod.acquia-sites.com/sites/default/files/2016-05/Res-StatementOnResilience_2.pdf.)
The manufacturing community also recognizes advanced materials are helping builders lead in their resiliency plans and goals. Chemical manufacturers are creating and enhancing various ‘ingredients’ that allow structures to better stand up against natural disasters, inclement weather, and the test of time. This article takes a deeper look at some of the latest materials chemical manufacturing companies are developing to increase building resilience.
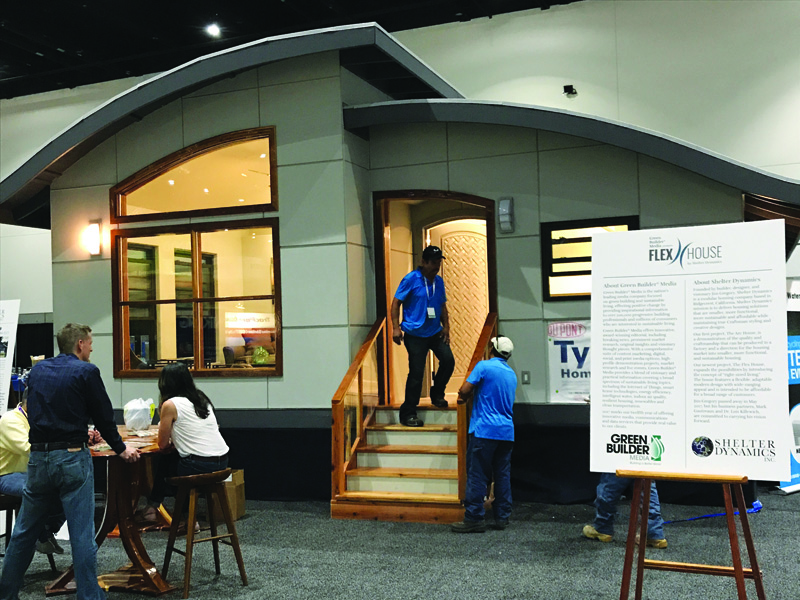
Photo courtesy Accella Polyurethane Systems
Chemistry of insulation
Over the past year, communities of all sizes have been impacted by devastating floods across the United States. Along with immeasurable suffering, these events can also cost billions in damages. (This statistic comes from www.ncdc.noaa.gov/billions/events/US/1980-2017. To read more about floodproofing measures, see the article “Time to Rethink Floodproofing: Recent Floods Have Shown Importance of Deployment Speed,” by Brian Shaw, CFM, in the August 2017 issue of The Construction Specifier.) Where properties are likely to be flooded, particularly in places close to rising sea levels or floodplains, insulation becomes incredibly important.
Certain types of wall insulation are formulated to increase a property’s resilience when faced with uncontrollable natural events. In the event a building is exposed to flood waters, the cavity or solid wall insulation with foam insulation may not be damaged, or will be less likely to need removal or replacement.
Sprayed polyurethane foam (SPF) was created through the work of chemists in the late 1930s. Chemist Otto Bayer, along with his colleagues, pioneered the chemistry of polyisocyanates—a technology used to create polyurethanes. This new material was so versatile it was employed for everything from shoes to cushions to industrial applications (even as a replacement for rubber during World War II). Since then, a variety of monomeric and polymeric isocyanates, polyethers, and acrylics have been introduced for use in the formulation of polyurethane. These components are mixed to form a rigid, cellular foam matrix. The resulting material is an extremely lightweight polymer with high-performing insulating properties.
A wall with sprayfoam has a higher racking strength, or ability to maintain its shape under duress, than a wall assembly without this insulation material. The bond SPF forms to the roof can increase a building’s resistance to wind uplift, which can help reduce damage experienced during periods of high wind. (For more information, check out Canadian Urethane Foam Contractors Association [CUFCA] research online at www.cufca.ca/research/SPF%20Research%20Report-Racking%20Strength-Council%20of%20BC%20Forests.pdf.) SPF helps seal as well as conserve energy, serving project teams striving to meet advanced energy codes and contribute toward green building certifications.
With respect to modern residential trends, the phenomenon of tiny homes, micro-apartments, and prefab cottages continues to grow. Even as square footage dwindles, the technology needed to ensure occupants stay warm in cold weather and, in some cases, that homes can be easily moved in the case of severe weather conditions, is increasingly important.