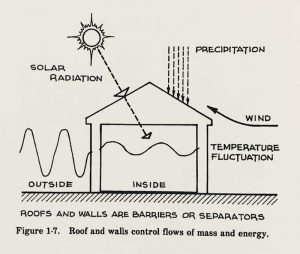
Images courtesy Roofs by Maxwell Baker, 1980, National Research Council Canada
Air barriers are systems comprising various materials and components. They are required by the International Energy Conservation Code (IECC) and work to control airflow and associated moisture. IECC also provides multiple compliance options utilizing a material’s or an assembly’s approach. Although not required by code, designers can specify compliance with whole building testing, which can holistically predict the in-situ air barrier system performance. Since they are restricting the movement of air, air barrier systems must be designed to withstand negative and positive pressures.
Vapor retarders manage vapor diffusion. They are not required by code in roofs, but designers may choose to specify them under certain circumstances, such as concrete roof decks where moisture can be an issue (read “Structural Concrete Decks, Vapor Retarders, and Moisture – Rethinking What We Know” by Helene Hardy Pierce and Joan P. Crowe). There are three classes of vapor retarders with different levels of permeance. A designer can calculate temperature gradients through an assembly to determine placement and need. Vapor retarders can act as air barriers and can be included in the air barrier system if detailed correctly. A good rule of thumb is warm air condenses on cold surfaces and vapor retarders are typically located on the warm side of an assembly. The challenge can be to determine the direction of vapor drive, especially in mixed climates. Understanding the project’s climate zone is one factor in determining whether a vapor retarder is recommended and where it should be installed.
Building codes
The International Code Council (ICC) publishes several codes applying to roof design (for purposes of this article, the 2015 and 2018 International Code Council [ICC] volumes are referenced. Each state and/or local municipality may amend the ICC and/or adopt their own codes. As such, this article is not intended to provide specific project code interpretation). The International Building Code (IBC), Chapter 1, “Scope and Application” notes the code “provides the minimum requirements to provide a reasonable level of safety, public health, and general welfare” through “safety to life and property”. IBC does not offer specific guidance on how buildings should perform after a hazard event beyond life-safety considerations. Chapter 13 of IBC says, “Buildings shall be designed and constructed per the International Energy Conservation Code (IECC). Chapter 1 of IECC provides requirements that construction documents be prepared by a registered design professional and that the insulation materials, R-values, air sealing details, and the building’s thermal envelope be included within the construction documents.”
Chapter 15 contains the majority of the roof-related requirements, including ANSI/SPRI ES-1 low-slope edge securement testing and compliance. Chapter 15 of the code also requires “roof coverings shall be designed and installed per code and manufacturer’s instructions. Parapet walls shall be properly coped, not less than the thickness of the parapet wall. Flashings shall prevent moisture/water from entering. Non-ballasted roofs, mechanically attached or adhered, shall be designed to resist wind load pressures for components and cladding.”
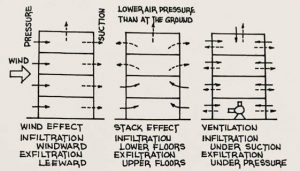
Roof decks and coverings must be designed for wind loads per Chapter 16 and Sections 1504.2 to 1504.4. Roof coverings shall resist impact damage per ASTM D3746, Standard Test Method for Impact Resistance of Bituminous Roofing Systems, D4272, Standard Test Method for Total Energy Impact of Plastic Films by Dart Drop, or Section 5.5 of Factory Mutual (FM) 4470, Single-Ply, Polymer-modified Bitumen Sheet, Built-up Roof (BUR) and Liquid Applied Roof Assemblies for Use in Class 1 and Noncombustible Roof Deck Construction. When designing a roof to better withstand impact hazards, consider the roof as a system and not just the attributes of individual components.
Chapter 16 of IBC provides the structural requirements for roofing systems, including the American Society of Civil Engineers (ASCE) 7, Minimum Design Loads for Buildings and Other Structures, wind pressure design needs. Wind design data shown on the construction documents includes risk category, wind exposure, internal pressure coefficient, and design wind pressures used for exterior cladding materials. There are opportunities for design professionals to create more durable roofing systems by making conservative selections (e.g. a more conservative risk category or wind exposure factor).
Functional recovery
Building functionality after a natural hazard event depends on the ability of the structure and the supporting infrastructure to withstand the disaster. In other words, even if a building remains intact after a storm, it may be difficult to occupy if the electrical grid is not functional. Current codes generally do not address continued functionality after a hazard event. However, ICC is a member of the Federal Emergency Management Agency (FEMA) Resilient National Partnership Network, a founding member of the U.S. Resiliency Council, and a signatory to the National Institute of Building Sciences (NIBS) Industry Statement on Resilience. An argument can be made to update codes to address emerging issues, such as extreme weather events, pre-disaster planning and post-disaster building functionality.
In their 2019 Natural Hazard Mitigation Saves report, NIBS found a monetary benefit is associated with communities adopting up-to-date building codes and designing beyond code minimums. According to the 2019 NIBS report:
- adopting model codes saves $11 per $1 spent;
- federal mitigation grants save $6 per $1 spent;
- private-sector building retrofit saves $4 per $1 spent;
- exceeding codes saves $4 per $1 spent; and
- mitigating infrastructure saves $4 per $1 spent.
However, front-end parties responsible for funding and construction appear to receive less benefit than end-users. This may be due to the economic investment required of front-end parties to implement resilience strategies. The report also documents non-monetary mitigation benefits, such as a reduction in deaths, nonfatal injuries, and post-traumatic stress disorder.
Excellent breakdown of high-performance roofing strategies! Informative content with practical insights. Thank you for enhancing our understanding of roofing dynamics.