by Scott Moffatt
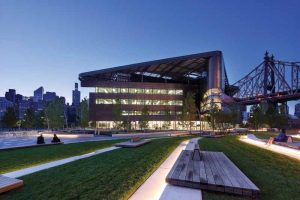
The coil and extrusion coatings industry is at a crossroads. Certification and testing bodies such as the American Architectural Manufacturers Association (AAMA) and ASTM have introduced stringent corrosion testing to better reflect real-world environments. Currently, there are discussions about raising industry standards for corrosion resistance in coastal and industrial environments. At the same time, architects, governments, municipalities, and also environmental organizations are demanding the elimination of materials on the Living Building Challenge’s (LBC’s) Red List, such as chromium and lead, traditionally used as corrosion inhibitors. This strategy, while well intentioned, has the potential to reduce corrosion performance of coated metal building components in hot, humid, salt-laden coastal areas and industrial environments.
The coil and extrusion coatings industry is committed to developing products offering increased corrosion performance and do not contain the Red List materials. While a comprehensive and cost-effective solution remains elusive, the industry is working to resolve these issues.
Corrosion and causes
The definition of corrosion is “the chemical or electrochemical reaction between a material, usually a metal, and its environment that produces a deterioration of the material and its properties.” Multiple factors can accelerate corrosion including:
- salt-saturated air and salt spray from oceans;
- high humidity;
- condensation (dew);
- sunlight;
- impact, freeze/thaw;
- time of wetness;
- industrialization (salt from de-icing roads, acid rain/smokestack emissions, and vehicle exhaust);
- dew, rain, and floods;
- birds and insects;
- fungus, bacteria, microbes, plant sap, and mildew;
- temperature and sunlight variation;
- dust, hail, lightning, gravel impact, abrasion, high-pressure spray;
- poor surface preparation;
- insufficient maintenance; and
- human perspiration.
Filiform corrosion
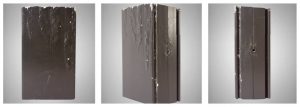
There are many forms of corrosion associated with the building market. One of the most prevalent is filiform corrosion. It mostly occurs on aluminum substrates. This type of corrosion begins when a substrate metal is exposed by a deep scratch, or when a cut edge enables moisture to penetrate beneath a metal coating.
Filiform corrosion requires relative humidity (RH) measuring between 40 and 90 percent. It spreads in streaks and creeps along the surface of the metal, causing the coating to lose adhesion and its aesthetic appeal. Filiform corrosion accelerates in warm temperatures, high humidity, elevated salt environments, and when metal is exposed by a raw or cut edge (Figure 1).
Aluminum extrusions and coil-coated materials are both susceptible to filiform corrosion because the metal edges are frequently exposed. Most aluminum extrusions are fabricated after coatings are applied, so when window frames are mitered, or fastener holes are drilled during installation, exposed edges may be left unprotected by the coating.
The vulnerability of exposed edges is also an issue for manufacturers applying paint to coil-coated stock. When paint is roll-coated onto the flat surface of the coil, edges are left uncoated. The same phenomenon occurs when painted coils are cut and fabricated into sheets. Fabrication bends in these applications may cause micro-cracking on the paint surface, opening another site for moisture penetration.
Galvanic corrosion
Galvanic corrosion occurs when electrochemically dissimilar metals share an electrically conductive path, enabling the ions from one of the metals to attack and oxidize the other. Applying a continuous paint film (coating) or inserting a gasket eliminating contact between two or more incompatible metals helps prevent this type of premature corrosion.